African cuckoo stands little chance with fork-tailed drongo
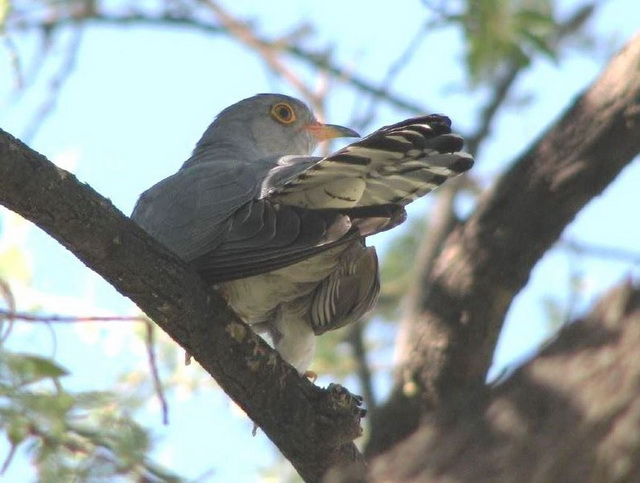
African cuckoo females lay their eggs in nests of fork-tailed drongos. They mimic drongo eggs very accurately – and yet drongos recognize more than 90 percent of cuckoo eggs, Jess Lund and colleagues show.
South of the Sahara lives the African cuckoo, Cuculus gularis, which, like the common European cuckoo, lays eggs in the nests of other bird species (one egg per nest) with the intention that foster parents will raise their chicks. The brood parasite targets only a few bird species, of which the fork-tailed drongo, Dicrurus adsimilis, is one of the most important.
But the cuckoo has hardly any success with this important host species, Jess Lund and colleagues show. The intended foster mother usually notices the deception because she has put a ‘signature’ on her own eggs for verification.
It is the outcome of the long evolutionary history that is shared by African cuckoo and fork-tailed drongo. There is a major conflict between both bird species, because the brood parasite fully depends on the services of the foster parent, and the burden on the foster parent is enormous.
Arms race
It starts with the fact that an African cuckoo female destroys a drongo egg after arriving to lay an egg in the nest of a fork-tailed drongo couple. The cuckoo chick finishes the job. It hatches first and pushes the drongo eggs out of the nest; if a chick happens to have hatched already, it is also thrown out. The foster parents lose their entire clutch. And they are busy for weeks with the demanding care of the foster chick.
This conflict with major interests created an arms race. The drongo learned to recognize cuckoo’s eggs and to reject them. In response, the cuckoo developed eggs that increasingly resembled drongo eggs. Currently, the mimicry is almost perfect: in the eyes of drongos, cuckoo eggs look exactly like drongo eggs.
Individual signature
Drongo eggs are hugely variable. The background colour ranges from white to reddish brown, and the eggs can be immaculate, speckled, or blotched. Between eggs of the African cuckoo, the same variation exists. The mimicry is excellent on population level, and the African cuckoo seems to be ahead in the arms race.
But in reality, the fork-tailed drongo is the winner.
That is because a drongo female consistently produces eggs with the same look. Each female has her own characteristic colour and pattern. She puts, as it were, a distinctive signature on each egg for verification: I laid this one. A cuckoo female lays eggs that fall within the drongo variation, but she lays them randomly. Chances are small that she lays an egg in the nest of a drongo female that produces exactly the same egg type. The cuckoo egg usually is aberrant.
Protected
Conducting experiments and using models, the researchers predict how likely it is that a fork-tailed drongo will recognize and reject an egg of the African cuckoo in her nest. And that is more than 90 percent! Without individual egg signatures, that chance would be much smaller. So, the strategy of drongos – great variation between clutches, great uniformity within clutches – is an excellent response to the almost perfect mimicry of cuckoos, protecting the drongo effectively against the brood parasite.
And so, the African cuckoo has little success with this host. A cuckoo’s egg seldomly is accepted. If you consider that about one in five drongo nests is lost during breeding, the brood parasite has an extremely low reproductive success. But apparently, that low success is enough for the species to survive.
Willy van Strien
Photo: African cuckoo. Alastair Rae (Wikimedia Commons, Creative Commons CC BY-SA 2.0)
Sources:
Lund, J., T. Dixit, M.C. Attwood, S. Hamama, C. Moya, M. Stevens, G.A. Jamie & C.N. Spottiswoode, 2023. When perfection isn’t enough: host egg signatures are an effective defence against high-fidelity African cuckoo mimicry. Proceedings of the Royal Society B, online 26 July. Doi: 10.1098/rspb.2023.1125
Stoddard, M.C., R.M. Kilner & C. Town, 2014. Pattern recognition algorithm reveals how birds evolve individual egg pattern signatures. Nature Communications 5: 4117. Doi: 10.1038/ncomms5117