Ringed caecilian-mother produces milk for her young
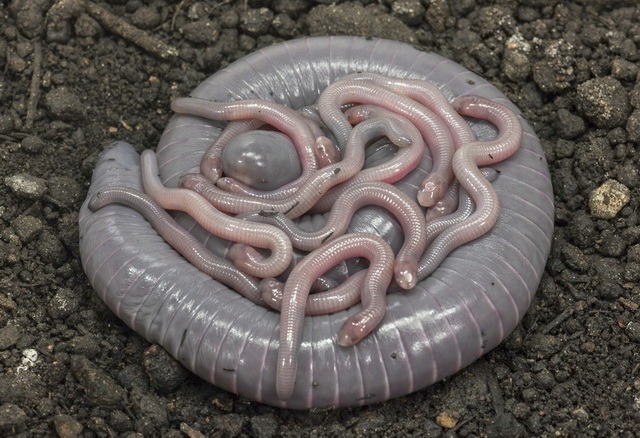
Females of the ringed caecilian secrete a fluid rich in fats and carbohydrates for their hatchlings, Pedro Mailho-Fontana and colleagues discovered. Like mammal’s milk.
Mammals suckle their young. This behaviour distinguishes them from other vertebrates: fish, amphibians, reptiles, and birds. Yet that distinction is not watertight, as a few bird species exist that produce a kind of milk to feed their young: some pigeons and doves, some flamingos, and the emperor penguin. And now, Pedro Mailho-Fontana and colleagues report that females of the ringed caecilian, an amphibian, feed their young with something that resembles mammalian milk. Thanks to this ‘milk’, the youngsters grow rapidly.
It is not surprising that this peculiar trait, which is easily observable, is only now coming to light. The biology of caecilians is poorly known because the animals live underground. Caecilians (Gymnophiona) form a third group within the amphibians, next to frogs & toads and salamanders. They have no legs, have reduced eyes, and are blind; they have two tentacles with which they find their way underground.
Spoon-shaped teeth
The caecilian in question here is the ringed caecilian (Siphonops annulatus). It is widespread in South America. The animal grows to over forty centimetres in length. A female lays eggs, on average ten at a time, in an underground round nest chamber. She is a devoted mother: she lays coiled up with the eggs on her body, and when the young have hatched, she stays with them for another two months; then the young are independent. Until that moment, she will not even leave for a brief time to find food for herself.
A female changes colour when she is attending hatchlings. Normally, ringed caecilians are bluish grey, but a mother turns whitish grey. It was already known that this colour is caused by fat droplets accumulating in her epidermis. Every few days, the young are allowed to scrape off that skin; for this purpose, they have spoon-shaped teeth in the lower jaw. They all do at the same time, ferociously; within ten minutes it is over, and peace returns until the mother’s skin is ready for consumption again. This ‘skin feeding’ occurs in more caecilian species.
But ringed caecilians hatchlings also get other food, Mailho-Fontana discovered: milk. He kept a number of animals in the lab and filmed their behaviour.
Oviductal milk
Hatchlings often assemble near their mother’s rear end, he observed. Further research revealed that glands in the mother’s oviduct walls produce a white, viscous fluid that emerges through the genital opening, the cloaca, several times a day. The stuff is rich in fats and carbohydrates.
The young imbibe it voraciously. This ‘milk’ is a more important source of nutrition than the mother’s skin, and it is thanks to the milk that the young grow very fast, the researchers think. Their weight doubles within a week after hatching. The mother, eating nothing, loses a third of her weight.
The mother releases milk when hatchlings touch her tail, often producing high-pitched sounds, which probably is begging behaviour. She then raises her body end vertically, and the hatchlings compete for a good place. On average, three of them drink at the same time until they are fully satiated.
Viviparous caecilian species in which the young drink milk in the oviducts before birth were already known. The discovery of oviductal milk in the egg-laying ringed caecilian was unexpected.
Willy van Strien
Photo: Ringed caecilian, female with young. ©Carlos Jared
Sources:
Mailho-Fontana, P.L., M.M. Antoniazzi, G.R. Coelho, D.C. Pimenta, L.P. Fernandes, A. Kupfer, E.D. Brodie Jr. & C. Jared, 2024. Milk provisioning in oviparous caecilian amphibians. Science 383: 1092-1095. Doi: 10.1126/science.adi5379
Jared, C., P.L. Mailho-Fontana, S.G.S. Jared, A. Kupfer, J.H.C. Delabie, M. Wilkinson & M.M. Antoniazzi, 2019. Life history and reproduction of the neotropical caecilian Siphonops annulatus (Amphibia, Gymnophiona, Siphonopidae), with special emphasis on parental care. Acta Zoologica. 100: 292-302. Doi: 10.1111/azo.12254
Wilkinson, M., A. Kupfer, R. Marques-Porto, H. Jeffkins, M.M. Antoniazzi & C. Jared, 2008. One hundred million years of skin feeding? Extended parental care in a Neotropical caecilian (Amphibia: Gymnophiona). Biology Letters 4: 358-361. Doi: 10.1098/rsbl.2008.0217